INTRODUCTION
Cancer is a fatal disease and is among the leading causes of death worldwide, making it imperative to find beneficial solution to defeat this public health menace [1]. Generally, cells in their normal health regulate the ratio of formation and destruction of cells in the body. This kind of homeostatic regulation is altered in cancer cells. Because cancer cells grow and divide more rapidly than normal cells, many anticancer drugs are made to kill growing cells thus the unwanted side effects. The development of multiple drugs resistance is a common clinical problem in the treatment of various cancers [2].
Despite the role currently played by synthetic chemistry in drug discovery and production method, the potential and the capacity of bioactive plants or their extracts to provide new research models and novel products for disease treatment and prevention is still almost unlimited [3,4]. The use of natural products as anticancer has a long history that began with traditional medicine. With previous successes from plant species or derivative of natural prototypes like vinblastine, vincristine, taxanes, etoposide and teniposide, increasing attention is being paid to naturally acquired compounds as new candidates [5, 6]
The growth inhibitory assay is a general bioassay that seems to be capable of detecting an anti-proliferative bioactivity of drug substances. Its commercially available, cheap, safe and easy to perform. It is simple and it requires little or no technology to make in the laboratories where appropriate human cell lines are not readily available. [7]. A number of studies have established the use of the seed growth inhibitory assay to screen plants commonly for anticancer and cytotoxic activity [8]. The radicle growth is recommended as an effective prescreen to existing cytotoxicity and antitumor assays [9]. The present study was conducted to evaluate the ant-proliferative activity of extracts of some selected Nigerian medicinal plants using the sorghum seed growth inhibitory assay. The plants were selected based on the information gathered from traditional healers.
MATERIALS AND METHODS
Collection and preparation of the experimental plants
The morphological parts of the plants (leaves and stem barks) were collected from the botanical garden of the National Institute for Pharmaceutical Research and Development (NIPRD). The leaves and the stem barks were air-dried and grinded into powder form using an electric milling machine. The powdered materials were kept in air tight container until required.
Phytochemical Screening of the Experimental Plants
These were carried out to test for the presence or otherwise of tannins, flavonoids, saponins, alkaloids, steroids and glycosides using standard methods [10].
Extraction of the Plants Material
About 150 g of each air-dried plant material of the each plant were macerated in glass jar and ina a ratio of 1:5 with the desired solvent for 3 days (72hours) at room temperature with occasional shaking and stirring. The contents of each jar were then filtered through a cotton plug and finally with a filter paper (Whatman. No 1). The filtrates were concentrated to dryness and maintained at 4◦C until required [11].
Experimental Plant (Sorghum bicolor)
Best quality sorghum bicolor seeds of uniform weight (35 ± 1 mg), obtained from Gwagwalada market in Abuja were used. The viability of the seeds was determined by their ability to remain submerged in water. Those that remained submerged in water were selected and dried for use. The seeds were sterilized by soaking in 70% alcohol solution for 2 min and washed with distilled running water. Twenty seeds were selected for each group (n = 20).
Determination of growth inhibitory effect of the extracts on guinea corn (Sorghum bicolor)
The twenty viable seeds selected were soaked in 15 ml of different concentrations of the plant extracts in small sample bottles for 24 hours. After 24 hours, each solution was poured into 9 cm wide Petri dishes laid with cotton wool of about 3mm thick sandwiched between two layers of 9 cm wide filter paper (Whatman No 1). The soaked viable seeds along with their respective solutions/concentrations were then transferred and spread on a corresponding labelled petri-dish and incubated in a dark environment favourable for plant growth. The lengths (mm) of the emerging radicles of the root and shoot of the seeds were measured at 24, 48, 72, and 96 hours. The positive and negative controls seeds were treated with equal volumes of 166.6 µg/ml methotrexate and distilled water respectively. Results were expressed as the mean growth inhibitory concentrations of the radicle.
Statistical Analysis
Graph pad prism version 6.02 was used to analyse data obtained and these were expressed as percentage growth inhibition and their IC50 were calculated.
RESULTS
Phytochemical screening
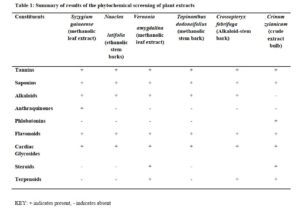
Click to view
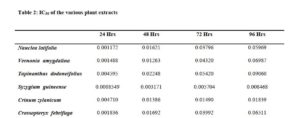
Click to view
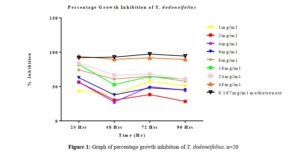
Click to view
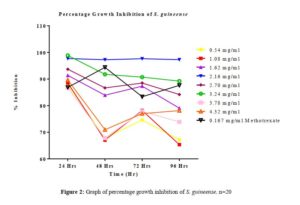
Click to view
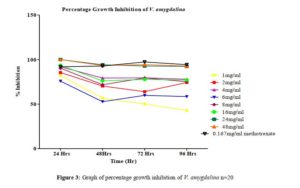
Click to view
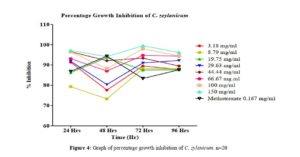
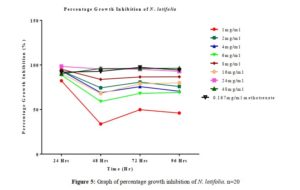
Click to view
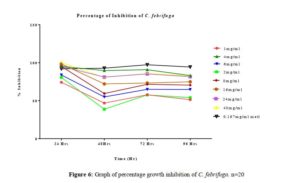
Click to view
DISCUSSION
This study evaluated the anti-proliferative potential of 8 extracts prescribed mostly by traditional healers for cancer therapy. The data obtained from the studies carried out on the plant extracts showed that they all possess a significant level of anti-proliferative effect. The seed growth inhibitory bioassay adopted to examine the probable anti-proliferative effect of the extracts is based on the principle that cytotoxic agents and shoot/radicle inhibition or growth reduction of seeds of plants such as cowpea, sorghum bicolor and maize have herbicidal or anticancer activity [10].
Cancer and tumor related ailments are characterized by uncontrolled cell proliferation in the body. Cellular proliferation depends on the rates of cell division and death and, thus, many anticancer drugs have been used to prevent cancer cell division in order to inhibit cancer cell proliferation. In vitro cytotoxicity assays can be used to predict human toxicity and for the general screening of chemicals [12, 13].
As previously reported by [14], depending on the test agent used and the cytotoxicity assay employed different cytotoxicity assays can give different results. Meristematic tissues of seeds have the tendency to proliferate in favorable conditions; the extent of this proliferation is reflected in the increase in the length of the radicles produced over 96 hrs in the control seeds. Although, other highly proliferative seeds such as cowpea (Vigna unguiculata), maize (Zea mays) can be used, Sorghum bicolor was found to be most convenient because of its relatively small size and low dormancy level.
The growth of both normal and cancerous cells is genetically controlled by the balance or imbalance of oncogene, protooncogene, and tumor suppressor gene protein products. Plant growth regulators (PGRs) are organic compounds, other than nutrients, that modify the physiological processes of plants [15]. Dormancy in the life cycle of plant or its part is the time at which active growth is temporary suspended in plant life cycle. It is controlled by bio-stimulators (e.g. gibberellins) and bio-inhibitors (e.g. abscisic acid) produced endogenously and help regulate plant metabolism. Seeds are essential part of the life cycle of any plant. Its germination can be exogenously and endogenously controlled by plant hormones [16].
To be effective, the seeds must absorb the PGRs into the embryo cells. For absorption of PGR to occur, the seeds were soaked in the test solutions for 24 hours. Soaking initiates water imbibition, softening of the seeds and germination. Growth rate increases with favorable temperature and moisture content, thus, the seeds were incubated to allow for such condition.
All tested extract produced remarkable dose and time-dependent anti-proliferative effect on radicle growth length of sorghum bicolor. Methotrexate 166.67 µg/ml which was used as the positive control elicits a more potent anti-proliferative effect as compared to the extracts. The criteria of cytotoxic activity of a crude extract according to the American National Cancer Institute (NCI) is an IC50 < 30 μg/ml [17]. With IC50 < 30 μg/ml, all evaluated extracts fall within the NCI criteria, thus are considered as having promising anticancer potential.
Methotrexate elicit its anticancer effect via disruption of polyamine synthesis by inhibiting dihydrofolate reductase which gives rise to reduced production of tetrahydrofolate and methyltetrahydrofolate, which are methyl donors in chemical reactions resulting in the production of methionine and S-adenosylmethionine and ultimately polyamines. Polyamines (PAs) are low molecular-weight organic polycations, displaying a broad biological activity in human and plants. The main PAs present in higher plants are putrescine (Put), spermidine (Spd) and spermine (Spm) [18-20]. In plants PAs exert a broad spectrum of biological activities such as the regulation of gene expression, signal modulation, cell proliferation and membrane stabilization [21-23]. These roles have been associated with the control of cell division, embryogenesis and root formation [24, 25]. Polyamine biosynthesis is up-regulated in actively growing cells, including cancer cells [26], therefore polyamine concentration as well as gene expression and activity of enzymes involved in polyamine biosynthesis, are higher in cancer tissues than in normal surrounding tissues [27]. Interestingly, these levels decrease after tumor eradication and increase after relapse [28], indicating that polyamines levels increased in case of cancerous growth.
These extracts may have also produced their anti-proliferative effect via inhibition of polyamine synthesis similar to methotrexate and in addition to other mechanisms yet to be understood. This effect could be a results of the phytochemicals contained in the extract that may be acting with the many plant regulators either singly or in synergy to elicit the inhibition of proliferation of cells.
These results demonstrate, for the first time the anti-proliferative properties of these extracts and also give further explanation to probable biochemical similarities in plant and animal cell proliferation as it relates to drug discovery and development.
ACKNOWLEDGEMENTS
The authors are grateful to the management of the National Institute for Pharmaceutical Research and Development for providing enabling environment for the conduct of the studies reported in this paper.
CONFLICT OF INTEREST
The authors declare no conflict of interest.
REFERENCES
[1]. Ayinde BA and Agbakwuru U. Cytotoxic and growth inhibitory effects of the methanol extract Struchium sparganophora Ktze (Asteraceae) leaves. Pharmacogn Mag. 2010; 6: 293-7.
[2]. de Martel C, Ferlay J, Franceschi S, et al. Global burden of cancers attributable to infections in 2008: a review and synthetic analysis. The Lancet Oncology 2012; 13: 607-615.
[3]. Fahey JW, Talalay P, Kensler TW. Notes from the field: green chemoprevention as frugal medicine. Cancer Prevention Research, 2012; 5 (2), pp. 179–188.
[4]. Lee KW, Bode AM, Dong Z. Molecular targets of phytochemicals for cancer prevention. Nature Reviews Cancer. 2011; 11 (3): pp. 211–218.
[5]. Kviecinski MR, Felipe KB, Schoenfelder T, de Lemos Wiese LP, Rossi MH, Gonçalez E, Felicio JD, Filho DW, Pedrosa RC. Study of the antitumor potential of Bidens pilosa (Asteraceae) used in Brazilian folk medicine. J Ethnopharmacol. 2008; 117: 69–75. doi:10.1016/j.jep.2008.01.017.
[6]. Hernandez-Aya LF, Gonzalez-Angulo AM. Adjuvant systemic therapies in breast cancer. Surgical Clinics for North America, 2013; 93: pp. 473–491.
[7]. McLaughlin JL, Chang C and Smith DI. Bench-top bioassays for the discovery of bioactive natural products: An update. In: Atta-ur-Rahman, editor. Studies in Natural Products Chemistry: Vol 9. Amsterdam: Elsevier Science Publishers, 1991; p. 383-409.
[8]. Wamidh H. Talib and Adel M. Mahasneh. Anti-proliferative Activity of Plant Extracts Used against Cancer in Traditional Medicine. Sci Pharm. 2010; 78(1): 33–45.
[9]. Ayinde BA, Omogbai EK and Ikpefan EO. Comparative cytotoxic and antiproliferative effects of Persea americana mill (lauraceae) leaf, stem and root barks. Niger. J Pharm Sci 2011; 10: 16-26
[10]. Harborne, J. B. Phytochemical screening methods: A guide to modern techniques in plants analysis. Chapman and Hal/. London, 1973; Pp: 47 – 74.
[11]. Ayinde BA and Agbakwuru U. Cytotoxic and growth inhibitory effects of the methanol extract Struchium sparganophora Ktze (Asteraceae) leaves. Pharmacogn Mag, 2010; 6: 293-297.
[12]. Clemedson C and Ekwall B. Overview of the final MEIC results. The in vitro–in vitro evaluation. Toxicology In Vitro 1999; 13: 657–663.
[13]. Scheers ME, Ekwall Ba, Dierickx JP. In vitro long-term cytotoxicity testing of 27 MEIC chemicals on HepG2 cells and comparison with acute human toxicity data. Toxicology In Vitro, 2001; 15: 153–161.
[14]. Weyermann J, Lochmann D, Zimmer A. A practical note on the use of cytotoxicity assays. International Journal of Pharmaceutics, 2005; 288: 369–376.
[15]. Wareing, P. F. Germination and dormancy. In Wilkins, M. B., eds. Physiology of plant growth and development. Chapter 17. McGraw-Hill, New York, New York. 1969.
[16]. Khan, A. A. Inhibition of gibberelllc acid-induced germination by abscislc acid and reversal by cytokinins. Plant Physiology 1968; 43: 1463-1465.
[17]. Itharat A, Houghton PJ, Eno-Amooquaye E, Burke PJ, Sampson JH, Raman A. In vitro cytotoxic activity of Thai medicinal plants used traditionally to treat cancer. J Ethnopharmacol. 2004; 90:33–38. doi:10.1016/j.jep.2003.09.014.
[18]. Bouchereau A, Aziz A, Larher F, Martin-Tanguy J. Polyamines and environmental challenges: recent development. Plant Science 1999; 140: 103–125.
[19]. Kuznetsov V, Radyukina NL, Shevyakova NI. Polyamines and stress: biological role, metabolism and regulation. Russian Journal Plant Physiology 2006; 53: 583–604.
[20]. Takahashi T, Kakehi JI. Polyamines: ubiquitous polycations with unique roles in growth and stress responses. Annals of Botany 2010; 105:1–6.
[21]. Tabor CW, Tabor H. Polyamines. Annual Review of Biochemistry 1984; 53: 749–790.
[22]. Cohen SS. A guide to the polyamines. Oxford: Oxford University Press 1998
[23]. Igarashi K, Kashiwagi K. Polyamines: mysterious modulators of cellular functions. Biochemical and Biophysical Research Communications 2000; 271: 559–564.
[24]. Kumar A, Taylora M, Altabella T, Tiburcio AF. Recent advances in polyamine research. Trends in Plant Science 1997; 2: 124–130.
[25]. Minocha R, Dale RS, Cathie R, Steele KD, Minocha SC. Polyaminelevels during the development of zygotic and somatic embryos of Pinusradiata. Physiologia Plantarum 1999; 105: 155–164.
[26]. Kubota S, Okada M, Yoshimoto M, Murata N, Yamasaki Z, Wada T, Imahori K, Ohsawa N, Takaku F: Urinary polyamines as a tumor marker.Cancer Detect Prev 1985; 8: 189-192.
[27]. Uehara N, Shirakawa S, Uchino H, Saeki Y: Elevated contents of spermidine and spermine in the erythrocytes of cancer patients. Cancer 1980; 45: 108-111.
[28]. Weiss TS, Bernhardt G, Buschauer A, Thasler WE, Dolgner D, Zirngibl H, Jauch KW: Polyamine levels of human colorectal adenocarcinomas are correlated with tumor stage and grade. Int J Colorectal Dis 2002; 17: 381-387.